The rostral cingulate cortex—the thick belt of tissue encircling the corpus callosum—plays a central role in contemporary models of emotion, pain, and cognitive control (https://tinyurl.com/hvld5yd). Work in these three basic domains has, in turn, profoundly influenced contemporary perspectives on more complex phenomena, including social cognition and a variety of neuropsychiatric disorders (https://tinyurl.com/neb48x9).
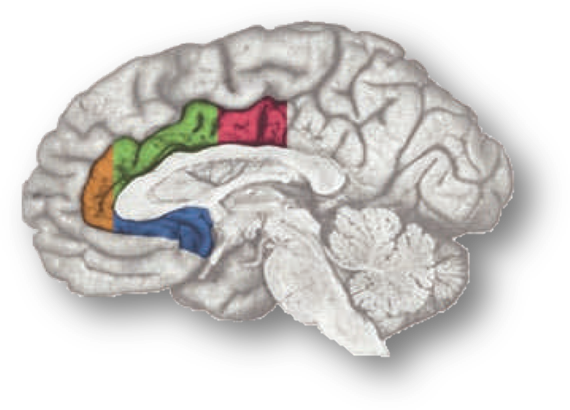
Despite this progress, the functional architecture and significance of activity in the rostral cingulate cortex remains enigmatic. We still don’t have a definitive answer to the question, What exactly does the cingulate ‘do’?
In a recent high-profile report (https://tinyurl.com/ofnxhtp), Matt Lieberman and Naomi Eisenberger make 3 extraordinary claims about the function of the dorsal or mid-cingulate cortex (‘dACC/MCC’), the region shown in green and magenta region in the accompanying figure:
1. We’re labeling it incorrectly. Lieberman and Eisenberger suggest that a substantial number of prior reports mis-labeled activation clusters lying in the more dorsal supplementary motor area (SMA) and pre-SMA as dACC/MCC:
“studies focused on the dACC are more likely to be reporting SMA/pre-SMA activations than dACC activations.”
2. We’re asleep at the wheel—Cognitive control does not reflect dACC/MCC. Lieberman and Eisenberger claim that standard lab assays of cognitive conflict and control—including the widely used Stroop, Eriksen flanker, Go/No-Go, and Stop signal tasks—do not produce robust activation in the dACC/MCC, contrary to more than a decade’s worth of imaging studies…
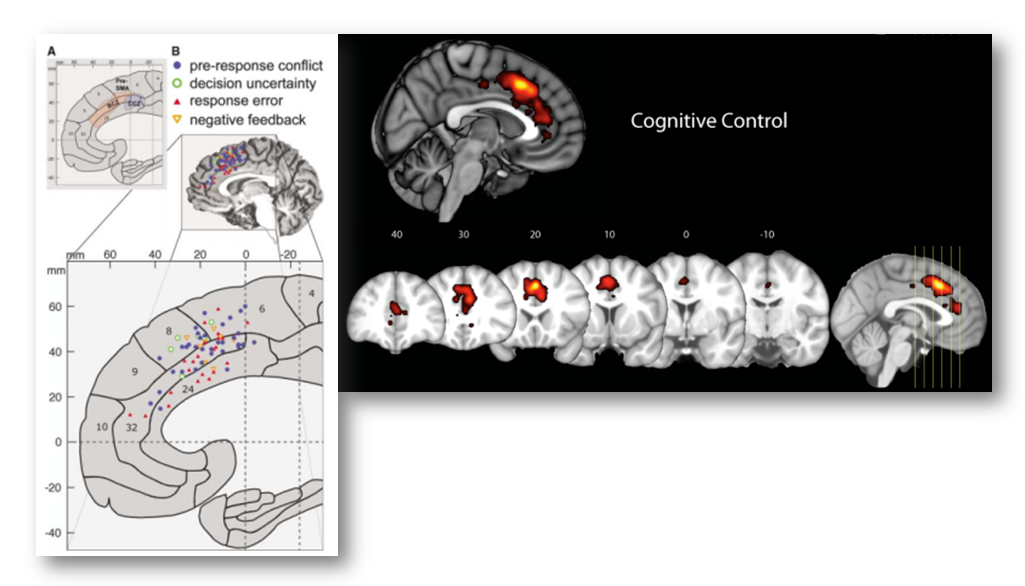
As they note,
“for many of the terms, the lion’s share of the activity is…in the [more dorsal] SMA or pre-SMA [i.e. the region depicted in green]…[Indeed] the forward inference maps for…“stop signal,” [and] “Stroop” each have a relatively modest footprint [in the dACC/MCC proper; the region depicted in blue].
3. The dACC/MCC is selective for pain. Based on automated meta-analyses of hundreds of brain imaging studies—performed using tools available at Neurosynth.org—Lieberman and Eisenberger argue that:
“The only psychological phenomenon that can be reliably inferred given the presence of dACC activity is pain…The conclusion from the Neurosynth reverse inference maps is unequivocal: The dACC is involved in pain processing. When only forward inference data were available, it was reasonable to make the claim that perhaps dACC was not involved in pain per se, but that pain processing could be reduced to the dACC’s “real” function, such as executive processes, conflict detection, or salience responses to painful stimuli. The reverse inference maps do not support any of these accounts that attempt to reduce pain to more generic cognitive processes. As seen in Fig. 3, a whole slew of such terms show little or no evidence of being likely candidates to explain the psychological bases of dACC activity. Although some of these functions might be instantiated in the [more dorsal] SMA or pre-SMA, they do not seem to be reliably related to dACC activity”
Naturally, such extraordinary, high-profile claims are bound to attract critics and skepticism. One of the most damning critiques comes in the form of a series of blog posts written by Tal Yarkoni, the developer of the software that Lieberman and Eisenberger used for their analyses (https://tinyurl.com/nvt3vlr and https://tinyurl.com/hhmgxvu). The posts are thoughtful, comprehensive, at times cranky, and well worth reading in their entirety, but for Yarkoni the bottom line is that:
“No, the dorsal anterior cingulate is not selective for pain….Lieberman & Eisenberger (2015) argue, largely on the basis of evidence from my Neurosynth framework, that the dACC is selective for pain. They are wrong. Neurosynth does not—and, at present, cannot—support such a conclusion. Moreover, a more careful examination of Neurosynth results directly refutes Lieberman and Eisenberger’s claims, providing clear evidence that the dACC is associated with many other operations, and converging with extensive prior animal and human work to suggest a far more complex view of dACC function.”
In particular, Yarkoni notes that voxels in the dACC/MCC are likely to be recruited in studies of fear, cognitive control, and conflict (see the following figure adapted taken from his post). If you’re curious, you can generate and download the same reverse inference maps yourself over at www.Neurosynth.org.
Given these observations, Yarkoni concludes that,
“In every single one of these cases, we see significant associations with dACC activation in the reverse inference meta-analysis. The precise location of activation varies from case to case…but the point is that pain is clearly not the only process that activates dACC. So the notion that dACC is selective to pain doesn’t survive scrutiny even if you use [Lieberman and Eisenberger’s] own criteria.”
For what it’s worth, I agree with Yarkoni’s conclusion, which he arrives at entirely based on a consideration of the statistics (see especially his second post, https://tinyurl.com/hhmgxvu).
A neuroanatomical perspective on the dACC/MCC
My own concern with Lieberman and Eisenberger’s study—one not addressed in either Yarkoni’s critique or Lieberman and Eisenberger’s thoughtful rebuttal (https://tinyurl.com/ptvhmjm)—has nothing to do with meta-analysis, Bayes, or statistics per se.
From my perspective, the crux of this story comes down to neuroanatomy—What exactly is the dACC/MCC?—Which voxels should be considered ‘in’ and which should be left ‘out’?
The answer to this fundamental question is central to Lieberman and Eisenberger’s claim that cognitive conflict and control engage the SMA/pre-SMA, whereas pain (and perhaps related states of fear and anxiety) engage the dACC/MCC. To foreshadow my conclusion, in the remainder of this post I will describe why Lieberman and Eisenberger’s approach to identifying the dACC/MCC is problematic and how this undermines their remarkable inferences.
My motivation—It’s about the cingulate, not Lieberman & Eisenberger
As an aside, I want to briefly address my motives for joining the public conversation about Lieberman and Eisenberger’s paper.
First, in the spirit of transparency, my colleagues and I have invested considerable effort trying to understand the rostral cingulate. If you’re curious, you can read more about our take here (https://tinyurl.com/hpqmkwn) and here (https://tinyurl.com/np3d3nb). In brief, we have hypothesized that the dACC/MCC represents a hub, where information about punishment, errors, conflict, and other kinds of negative feedback is integrated and used to bias or control our thoughts, feelings, and actions in the face of uncertainty about actions and motivationally significant outcomes, such as punishment. One of the motivations for this hypothesis was evidence from imaging studies and neuronal recordings that tasks that elicit negative affect (e.g. fear), physical pain, or the need for increased cognitive control all recruit an overlapping territory in the anterior dACC/MCC.
Naturally, Lieberman and Eisenberger’s claim that dACC/MCC activation is specific to pain seems to run counter to the idea that this key brain region is consistently recruited across diverse psychological states. But, in their rebuttal to Yarkoni, Lieberman and Eisenberger clarify their conclusions in ways that ameliorate some of my initial concerns, arguing for example that they simply meant that “pain is a more reliable source of dACC activation than the other [i.e. cognitive] terms of interest” and that the dACC/MCC is, in fact, exquisitely sensitive to negative affect: “We have long posited that one of the functions of the dACC was to sound an alarm when certain kinds of conflict arise. We think the dACC is evoked by a variety of distress-related processes including pain, fear, and anxiety.” Still, their claims that the dACC/MCC is only weakly sensitive to demands for increased cognitive control was at odds with my own understanding of the literature and my interpretation of the maps that I could generate with a few button clicks in Neurosynth. So, I wanted to understand why we were reaching such different conclusions.
My second motive for entering this debate is more substantial and, hopefully, generative. I want to understand what the dACC/MCC does and leverage that understanding to guide the development of more effective interventions. My concern is that there is widespread misunderstanding about what constitutes the dACC/MCC. For example, in their blog posts, Lieberman, Eisenberger, and Yarkoni all seem to agree that the cluster in this next figure does not lie in the dACC/MCC—but based on the evidence that I review below, I believe that this is incorrect.
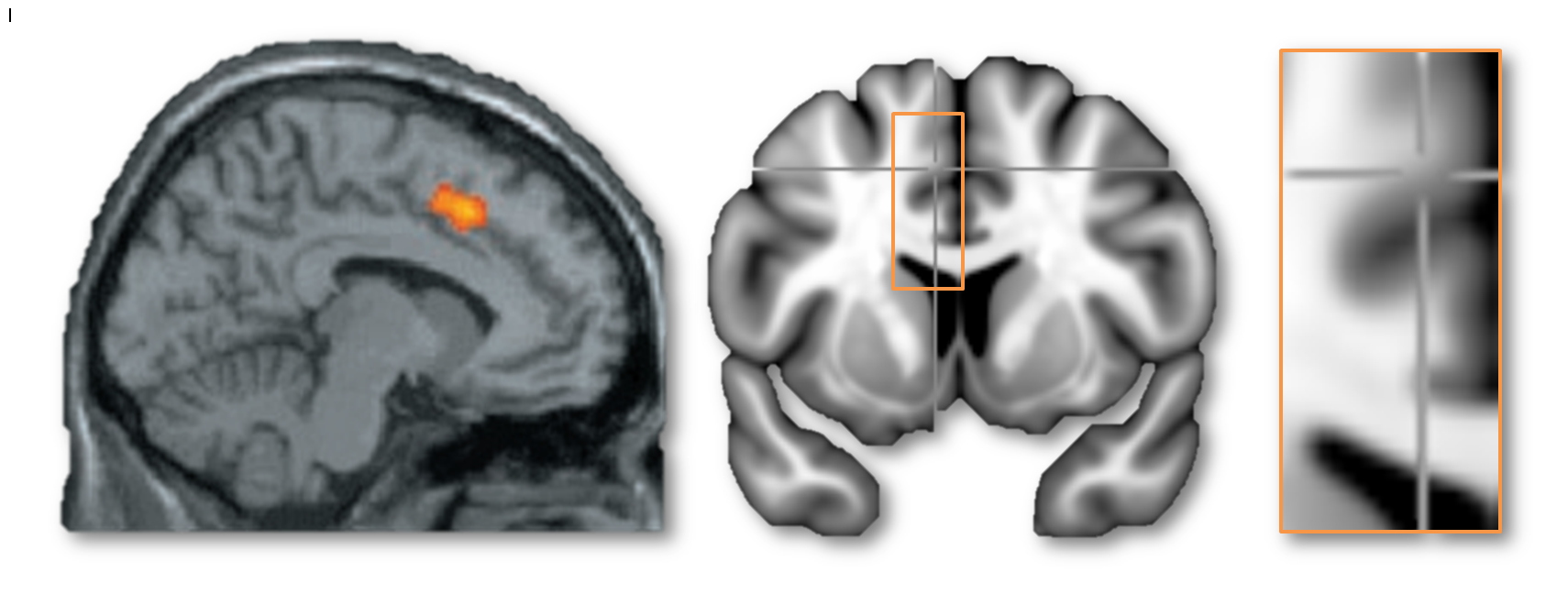
I hope that by reviewing what is known about the anatomy of the dACC/MCC and articulating some simple, concrete methodological recommendations for future imaging studies, it will accelerate our understanding; that the graduate students, post-docs, and PI’s who attempt to build on the published literature will adopt methods that permit stronger inferences about what the cingulate does.
Lieberman and Eisenberger fail to address individual variation in anatomy
Lieberman and Eisenberg describe the dACC/MCC as the
“section of the cingulate that sits above the corpus callosum…the cingulate sulcus is the dorsal boundary of the dACC. Above this sulcus are the supplementary and pre-supplementary motor areas (SMA and pre-SMA), which…are contained within the superior frontal gyrus.”
This is the only description in the paper of how they prescribed their dACC/MCC region-of-interest (ROI; the blue region in the next figure). Fortunately, we can reverse engineer what they probably did. Close inspection of their figures suggests that the dACC/MCC ROI was manually prescribed on the Colin27 brain (https://tinyurl.com/o55nffe; left panel below), which is simply the average of 27 anatomical scans of a single adult man (the eponymous Dr. Colin J. Holmes, shown in the right panel) in standard stereotaxic space (https://tinyurl.com/q2jqgeu).
What’s wrong with relying on the brain of one Canadian scientist for drawing the dACC/MCC ROI? After all, they’ve given us Hebb, Penfield, the MNI, and Tim Horton’s right? Well, as the developers of the Colin27 template note:
“this dataset was not originally intended for use as a stereotaxic template… As a single brain atlas, it did not capture anatomical variability and was, to some degree, a reversion to the [outdated] Talairach approach.”
In other words, Lieberman and Eisenberger conducted meta-analyses based on hundreds of brain imaging studies, collectively encompassing thousands of individual subjects, each with their own idiosyncratic brain.Each one of those thousands of brains was ‘warped’ (i.e. ‘registered’ or ‘normalized’) to a standard brain template by the original investigative teams. This standardization not only allowed those investigators to compute group statistics, it‘s also what makes it possible to conduct large-scale meta-analyses in Neurosynth or other software packages (http://brainmap.org)—all of the activation peaks are reported in the same 3D Cartesian space (more or less; https://tinyurl.com/hjqy6r5).
The problem is that the warping process is imperfect; there are errors in how well each brain is registered to the template, especially in studies relying on earlier generations of warping techniques (like the well-known Talairach and Tournoux stereotaxic technique; https://tinyurl.com/o83ksuk). So, it’s not appropriate to think of features of the brain—like the dACC/MCC—as a crisp point or line, as in the left panel of the following figure.
Given registration errors across many, many individual brains, it’s better to think of these features in a more probabilistic way (as in the right panel above), which is why most investigators (and NeuroSynth) use brain templates that were generated by averaging across tens or hundreds of warped brains, as in the popular MNI152 template shown below (https://tinyurl.com/zgjv4a3).
In sum, the Colin27 brain template (apparently) used by Lieberman and Eisenberger conveys a false sense of precision about the sulcus separating the cingulate from the overlying SMA/pre-SMA. But this is only the first part of what is really a compound problem. As it turns out, we probably do not want to rely on just the cingulate sulcus, let alone just Colin’s cingulate sulcus.
Lieberman and Eisenberger define the dACC/MCC in an arbitrary manner
It’s worth pausing for a moment and considering how the rostral cingulate has traditionally been defined on the basis of invasive studies of cellular morphology and chemistry. The neuroanatomist Korbinian Brodmann (https://tinyurl.com/glex9gp) first defined the rostral cingulate as the complex formed by architectonic areas 24, 32, 25, and 33. Of these, areas 24, 32, and 33 (marked with red circles below) constitute the subdivision lying dorsal to the corpus callosum (dACC/MCC).
As shown in the next figure, much of the cortical gray matter that makes up the dACC/MCC lies buried in sulci and is not visible in sagittal images. For brains—like Colin’s or that shown in the next figure—that only have a single cingulate sulcus, Lieberman and Eisenberger’s dACC/MCC ROI probably does a pretty good job. As shown in upper right panel (https://tinyurl.com/hvld5yd), as long as they included the voxels located in the dorsal bank of the cingulate sulcus (shown in purple), their ROI would encompass all of the dACC/MCC (i.e. areas 33, 24, and 32).
Whether that’s the case is unknown; the ROI protocol is not described in Lieberman and Eisenberger’s report and they do not show any coronal images. It’s not really any more clear in their rebuttal to Yarkoni, although it looks like they may be missing some of the dorsal bank (technical aside: the green line demarcating the dACC/MCC region is not a genuine 3D ROI and is clearly for illustrative purposes only).
Lieberman and Eisenberger fail to account for variation in cingulate anatomy, revisited
If you accept the historical definition of dACC/MCC described above—dACC/MCC = areas 24′, 32′, and 33’—then the real problem comes from the fact that there are marked individual differences in the gross anatomy of the rostral cingulate, differences that often push area 32′ into the gray matter overlying the cingulate sulcus.
As my colleagues and I noted in a 2011 review (https://tinyurl.com/hvld5yd cited by Lieberman and Eisenberger):
“there is considerable variability in the paracingulate sulcus (PCgS), a tertiary sulcus that is present in about one-half of the population and more prominent in the left hemisphere (see the following figure, part a). The presence of this sulcus exerts a strong impact on the layout and relative volume of the architectonic areas comprising [dACC/MCC]…In particular, area 32’, which is otherwise found in the depths of the cingulate sulcus (CgS), expands to occupy the crown of the external cingulate gyrus (ECgG; the ‘superior’ or ‘paracingulate’ gyrus)…[In short] the size and spatially normalized location of the [dACC/MCC] can vary substantially across individuals…Accounting for such individual differences may permit a clearer separation of intermingled affective, nociceptive and cognitive processes [in this region]”
This is not a rare or isolated effect. As shown above, Brodmann himself depicts area 32 as lying between the cingulate (orange arrows) and paracingulate sulci (red arrows).
Likewise, Vogt and colleagues emphasize that,
“Area 24c is located on the dorsal bank of the cingulate gyrus and the ventral bank of the superior cingulate gyrus [often termed the ‘paracingulate’ gyrus] and it extends rostral to the genu but not into subgenual ACC…Area 32 is located mainly on the [paracingulate gyrus]…”
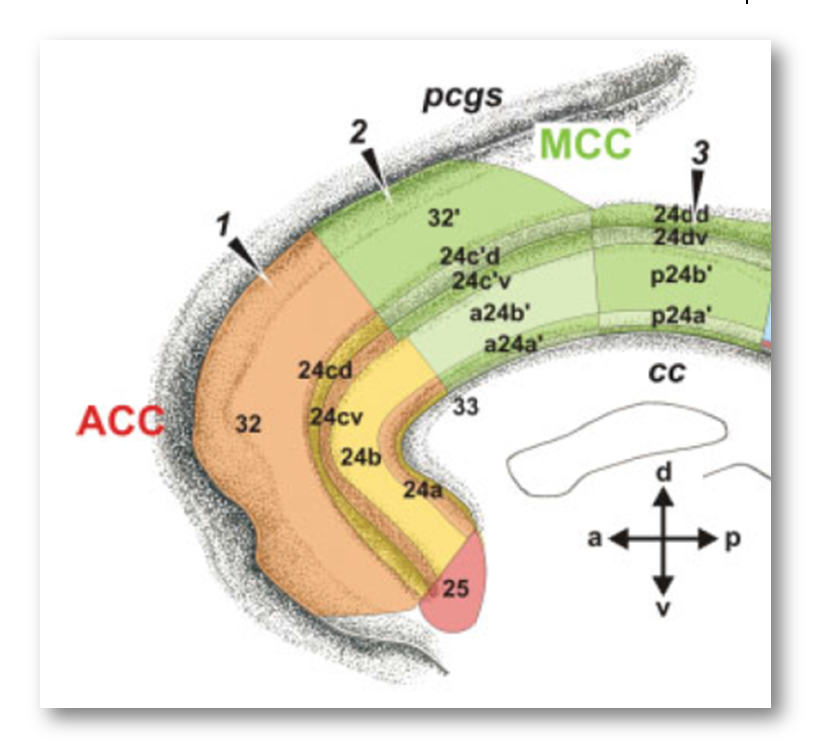
From this perspective, Lieberman and Eisenberger’s blue dACC/MCC blob, which stops at Colin’s cingulate sulcus, probably omits a substantial portion of area 32’ for many of the individual brains that underlie their meta-analyses. This is particularly problematic because cognitive neuroscientists generally regard area 32’ as the cingulate region most closely involved in cognitive conflict and control (https://tinyurl.com/p8krylv).
Lieberman and Eisenberger acknowledge these kinds of concerns in the Supplement to their report:
“There is substantial sulcal variability within the dACC….The critical question, then, is whether effects we have designated as outside the dACC…might be in the dACC after all.”
But quickly dismiss them:
“There is no way to definitively rule out this possibility in the current study. Neurosynth doesn’t have coding for individual participant morphology. Moreover, almost no fMRI studies account for these individual differences. The vast majority of fMRI studies overlook most individual differences in neuroanatomy and depend on the probabilistic neuroanatomy averaged across a group of participants and then on standard atlases that typically don’t take these individual differences into account.”
This is partially true—insofar as only a handful of fMRI studies have explicitly modeled individual differences in dACC/MCC gross anatomy (e.g. https://tinyurl.com/nsdjo3n and https://tinyurl.com/nch92eo)—but it is also deeply misleading.
In fact, as we have just seen there are at least two simple, concrete ways to begin to address variation in cingulate anatomy:
- Use a probabilistic template, like the MNI152, that ‘bakes in’ information about warping (registration) error and variation in cingulate neuroanatomy
- Define the dACC/MCC ROI in a way that is consistent with well-established macroscopic anatomical borders; like Brodmann, Vogt, and many other investigators include the paracingulate (‘superior cingulate’) gyrus as well as voxels located in the depths of the cingulate and paracingulate sulci.
Sound like too much work? In fact, the ROI can be defined automatically using freely available probabilistic atlas labels. For example, the Harvard-Oxford atlas (https://tinyurl.com/h3m8b8w), which is included with many imaging software packages, is based on the overlap of labels generated from high-resolution anatomical scans obtained from 37 men and women of varying ages.
Applying these simple recommendations yields a very different, rather boring conclusion
Let’s see what happens when you use a probabilistic template, a probabilistic atlas, and include all of the dACC/MCC. Here is the reverse inference map reported by Lieberman and Eisenberger for studies of ‘conflict.’
The white traces indicate their dACC/MCC (lower region) and the SMA/pre-SMA (upper region) ROI’s overlaid on Colin’s brain. Uh, oh! It looks like most of the ‘conflict’ cluster (in blue) lies in the SMA/pre-SMA (upper white region).
Here is the same map re-created using Neurosynth and overlaid on the MNI152 brain at FDR q < .01 (MNI coordinates = 7/26/35). Now it looks like the cluster (in red; colors are arbitrary) is squarely centered on the cingulate sulcus.
Here’s another view of the same map thresholded at Z > 7.0 to emphasize local maxima. The upper 3 panels are centered at MNI coordinates 7/26/35, which corresponds to a 46% probability of lying in the paracingulate gyrus and a 36% probability of lying in the anterior division of the cingulate gyrus in the Harvard-Oxford probabilistic atlas. The lower panel is centered at 7/15/41 (52% paracingulate, 28% anterior cingulate gyrus, and 1% supplementary motor cortex). The Harvard-Oxford atlas indicates that there is a very low probability that these maxima lie in the superior frontal gyrus or supplementary motor cortex, contrary to a SMA/pre-SMA label. Inspection of the coronal images (on the far left side of the figure) indicates that the local maxima lie in the dorsal bank of the cingulate sulcus, which is consistent with the current consensus about the role of area 32’ in monitoring cognitive conflict. My intuition is that Brodmann, Vogt, and most other investigators would label these maxima as dACC/MCC. In contrast, if we project these two points onto the sagittal plane (x=3) used in Lieberman and Eisenberger’s report and display them on Colin’s brain (right-most panels), we would draw the erroneous conclusion that they fall in the overlying SMA/pre-SMA.
Importantly, this conclusion is not specific to ‘conflict.’ A number of other, closely related reverse-inference maps in Neurosynth show similar effects, including ‘Control’ (8/8/42; 44% cingulate gyrus anterior division, 20% paracingulate, 11% supplementary motor), ‘Errors’ (4/26/36; 63% paracingulate and 37% cingulate), ‘Stop Signal’ (6/32/34; 73% paracingulate, 9% cingulate gyrus anterior division, and 1% superior frontal gyrus), and ‘Stroop’ (6/22/38; 55% paracingulate gyrus and 27% cingulate gyrus anterior division).
In short, when probabilistic methods and longstanding definitions of the dACC/MCC are employed, we arrive at the much less extraordinary claim that tasks that demand top-down control engage the dACC/MCC.
Conclusions
So what does all of this neuroanatomy mean for Lieberman and Eisenberger’s three key claims and for past and future research more generally?
- We’re mostly labeling it correctly. Lieberman and Eisenberger claim that a substantial number of prior reports mis-labeled activation clusters in the SMA/pre-SMA as dACC/MCC. This is wrong. It reflects the inappropriate use of Colin’s brain and an idiosyncratic definition of dACC/MCC that excludes regions of the brain that have been considered part of the dACC/MCC for over a century. In all likelihood, a substantial portion of area 32’ was excluded from Lieberman and Eisenberger’s dACC/MCC ROI. As a consequence, voxels lying in this region were erroneously attributed to the more dorsal SMA/pre-SMA.
- We’re not asleep at the wheel. Lieberman and Eisenberger claim that standard cognitive conflict tasks do not produce robust activation in the dACC/MCC. As I have shown here, this claim is wrong; it reflects a failure to properly address individual differences in cingulate neuroanatomy. When even a minimal effort is made to address this variation—by simply employing probabilistic templates and atlases—the results are consistent with the widespread belief that cognitive conflict tasks robustly engage the dACC/MCC in the probable location of area 32’.
- The dACC/MCC is not selective for pain. Based on the analyses described in Yarkoni’s rebuttal and the concerns that I have outlined here, I believe that Lieberman and Eisenberger’s central claim is also wrong. In fact, the weight of the evidence—from fMRI studies and otherwise—is consistent with the idea that the dACC/MCC is recruited by a range of emotional, nociceptive, and cognitive challenges, as we and many others have repeatedly emphasized. It may well be the case that emotion, pain, and cognitive control are segregated at a finer level of anatomical resolution (e.g. deep vs. superficial or rostral vs. caudal or sulcal vs. gyral subdivisions of dACC/MCC; as we have noted https://tinyurl.com/hvld5yd), but this was neither the aim nor the conclusion of Lieberman and Eisenberger’s remarkable report.